Chirality, two objects being mirror images of one another, is a widespread phenomenon at all scales in nature, down to the molecular level. This is important, as chirality in pharmaceutical molecules results in their enantiomers usually having markedly different biological properties. Therefore, separating enantiomers from racemic mixtures and obtaining adequate enantiomeric purity for APIs has always been a priority for the pharmaceutical industry.
While several techniques are available for chiral separation, crystallization is most often the preferred method in industry, as it is generally more cost-effective and scales better, compared to other methods, such as chromatography. Interest in chiral crystallization has spiked in the past decades, with the invention of deracemization methods, such as abrasion induced Viedma ripening and dynamic preferential crystallization, which in theory allow for 100% enantiomeric yield.
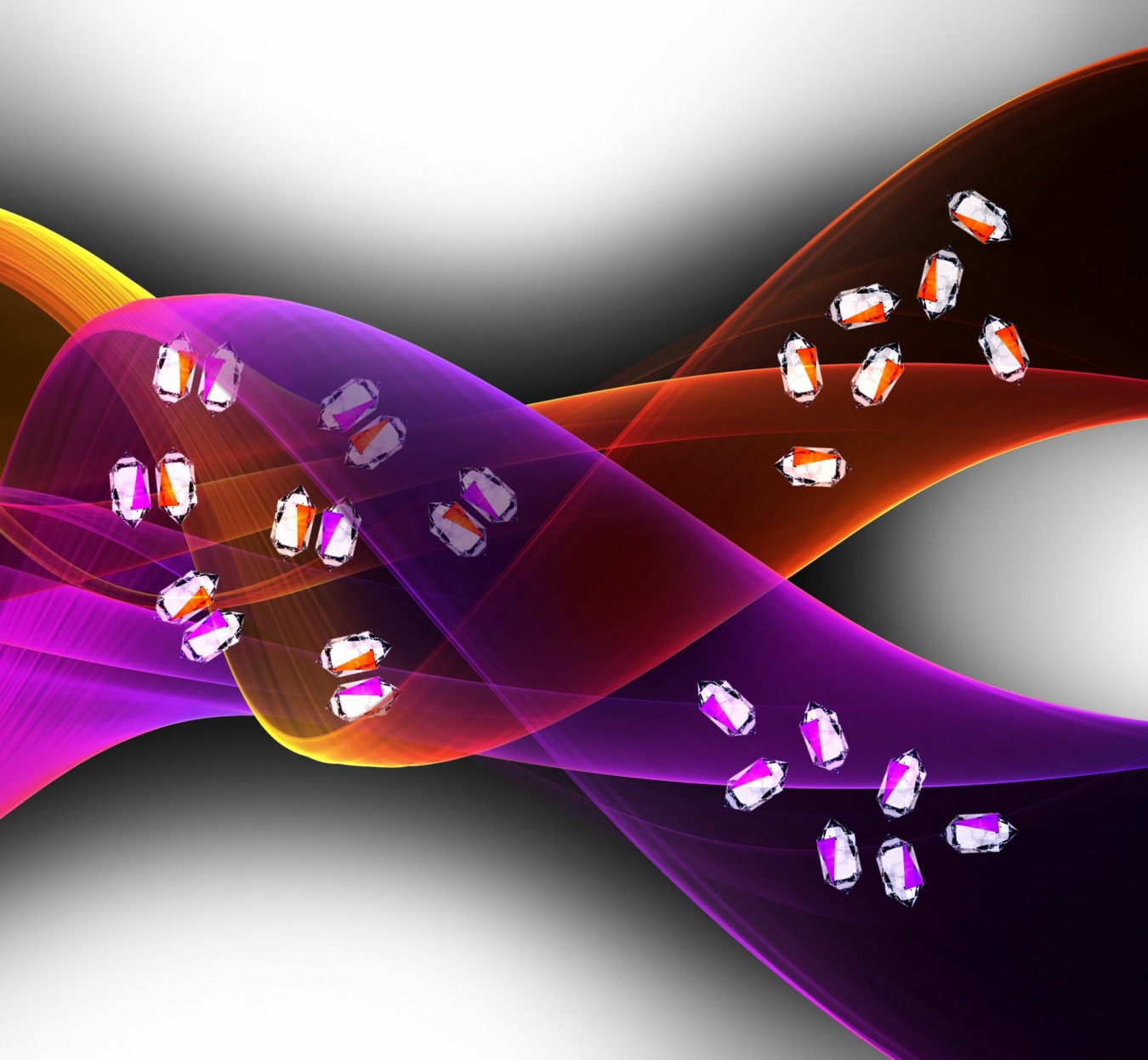
Nevertheless, all available separation techniques, both crystallization and chromatography based, rely on the same foundational solubility information. A good example of this is the continuous chiral resolution process using membrane ultrafiltration and crystallization developed by Joop ter Horst’s group. Here, bovine serum albumin is used as a chiral selector for enriching the enantiomeric excess of certain amino acids, which then crystallize. The Crystal16 device was used for determining the solubility information. [1]
A second piece of key information for process development is understanding and characterizing how a chiral molecule crystallizes, as a conglomerate, racemate or solid solution. One such example is the fundamental study of Harfouche et al, where the nucleation rates of racemic and enantiopure histidine are investigated. In this study, the Crystal16 proved invaluable for performing the induction time experiments required to calculate the experimental nucleation rates. [2]
The Crystal16 has proved an invaluable tool for developing chiral separation and deracemization methods in 2021. Mazzotti’s group investigated the chromatographic separation and deracemization of an amino acid derivative in a SMB process. A modified version of the Crystal16 was used to determine the crucial racemization rates for designing the process. [3]
A second example of novel deracemization methods was described by Guillot et al., where co-crystallization spontaneous induced deracemization. The process relies on the preferential formation of the R-S cocrystal compared to the S-S cocrystal of 4,4-dimethyl-1-(4-fluorophenyl)-2-(1H-1,2,4-triazol-1-yl)-pentan-3-one (BnFTP) and 3-phenylbutyric acid (PBA). Crystal16 solubility data was used to first select the process solvent and then to determine the solubility difference between the two diastereomeric cocrystals. [4]
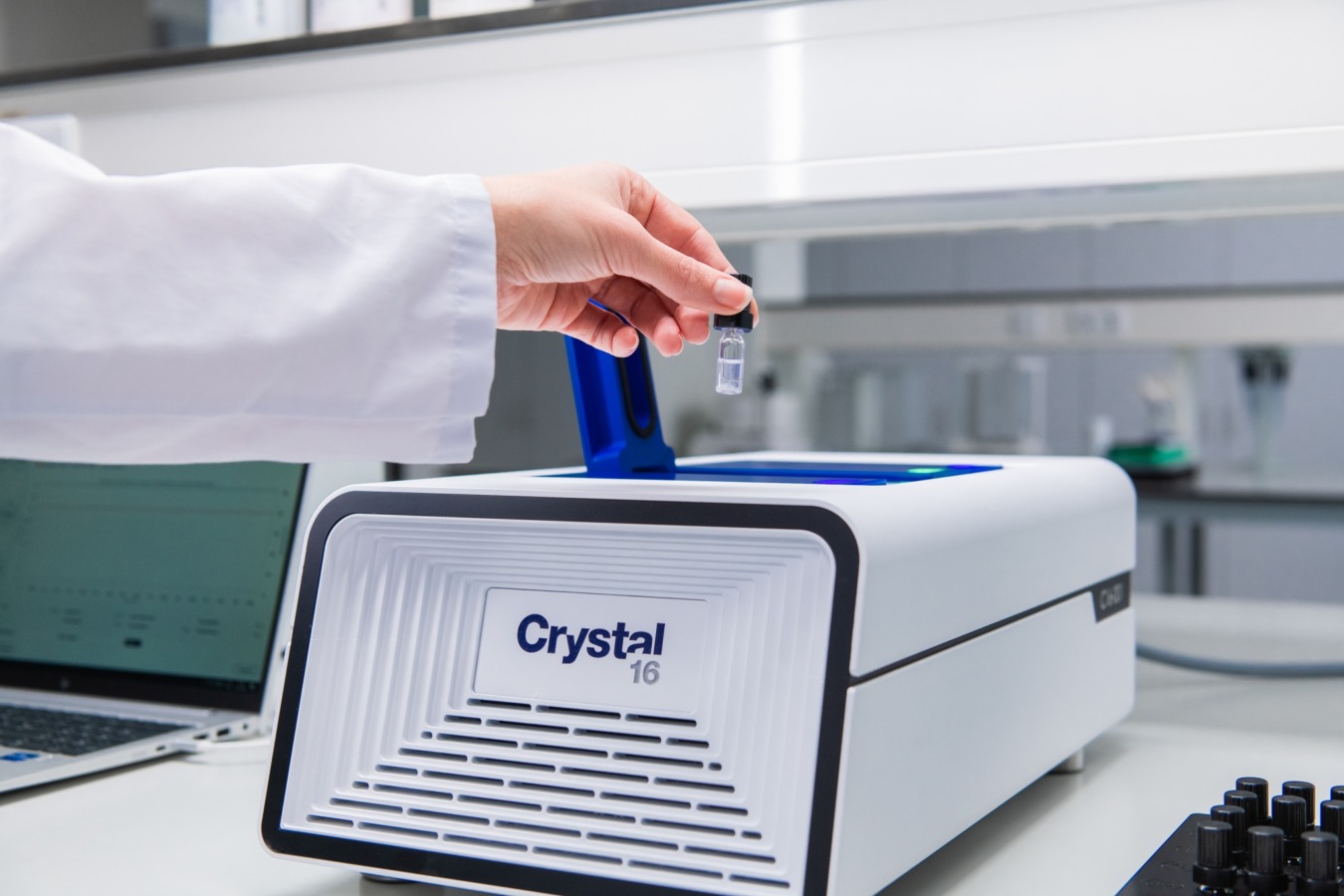
In another important development, Flood’s group investigated the epimerization of threonine to allothreonine using an immobilised enzyme, amino acid racemase, and temperature cycling. In this case, the larger CrystallinePV instrument was used in a preparative way by allowing the one-pot epimerization reaction to take place under controlled temperature and stirring conditions. 5
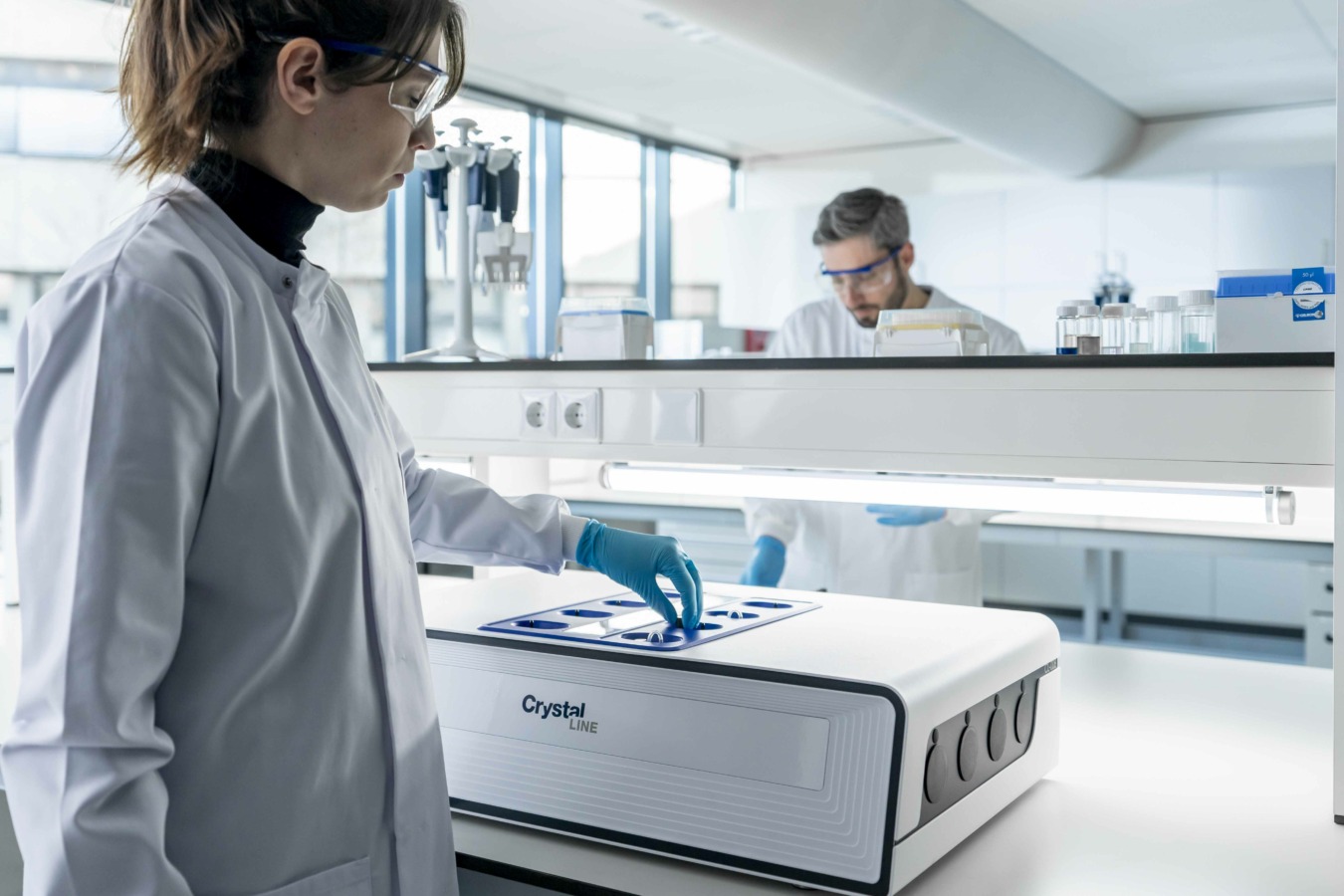
References:
- Jose Capdevila-Echeverria, Jiayuan Wang, Richard Lakerveld, Joop H. ter Horst, Journal of Membrane Science, 2021, 632, 119359, https://doi.org/10.1016/j.memsci.2021.119359
- Lina C. Harfouche, Simon Clevers Gérard Coquerela and Ivo B. Rietveld, CrystEngComm, 2021,23, 8379-8385 DOI https://doi.org/10.1039/D1CE01199E
- Francesca Breveglieri, Tuvshinjargal Otgonbayar, and Marco Mazzotti Industrial & Engineering Chemistry Research 2021 60 (29), 10710-10719 DOI: 10.1021/acs.iecr.1c00679
- Michael Guillot, Joséphine de Meester, Laurent Collard, Olivier Riant, and Tom Leyssens Organic Process Research & Development 2021 25 (4), 884-891 DOI: 10.1021/acs.oprd.0c00538
- Kritsada Intaraboonrod, Andreas Seidel-Morgenstern, Heike Lorenz, and Adrian E. Flood Crystal Growth & Design 2021 21 (10), 5641-5650 DOI: 10.1021/acs.cgd.1c00484