A look back at applications with Crystalline in 2023
The Crystalline is a versatile lab system featuring 8 individual reactors, each capable of temperatures ranging from -25 °C to 150 °C. It features integrated analytical techniques like turbidity, particle view imaging, Raman spectroscopy, and AI-driven shape and size analyses for particle size distribution. Furthermore, its ergonomic and user-friendly design is ideally suited for robotic applications.
Crystalline's widespread use in various research and development projects during 2023 underscores its effectiveness and utility. Here we share with you some of its different applications published last year.
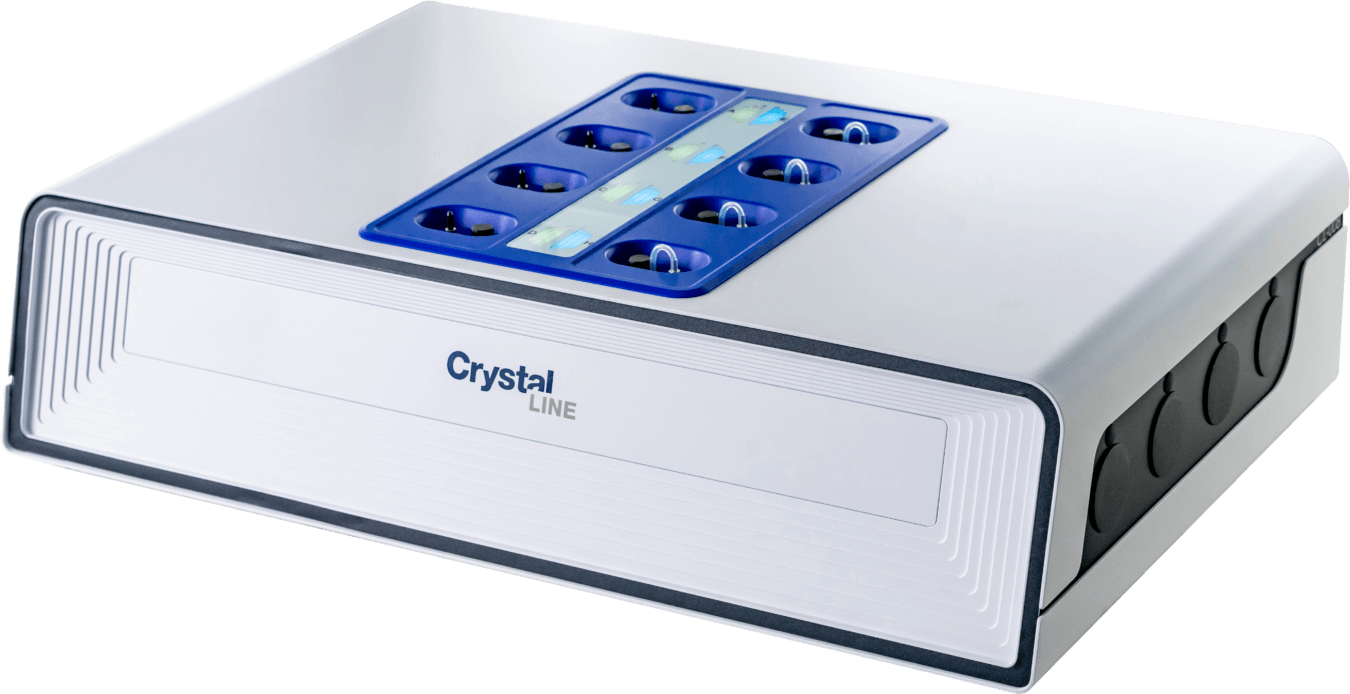
Using Crystalline to conduct metastable zone width (MSZW) studies
Nandi et al. [1] demonstrated that the Crystalline instrument, equipped with a 3.5 ml solution volume in a glass reactor and a 3-blade downflow impeller, is an optimal platform for conducting MSZW (Metastable Zone Width) studies. Their innovative use of a combined polythermal and antisolvent addition approach enabled a deeper understanding of solubility and nucleation kinetics in liquid antisolvent crystallization (LASC). This methodology allows for extracting kinetic information under high driving forces, significantly enhancing the accuracy of predicting LASC processing conditions.
Using Crystalline to assess primary and secondary nucleation and growth kinetics
Cashmore et al. [2], at the University of Strathclyde, Glasgow, conducted small-scale experiments using Crystalline, which features in-situ imaging for crystal counting and sizing. They focused on quantifying nucleation and growth kinetics under isothermal conditions for seeded and unseeded crystallization. Their study detailed an efficient experimental workflow for rapidly assessing primary and secondary nucleation and growth kinetics, exemplified by the cooling crystallization of α-glycine from aqueous solutions in magnetically agitated vials.
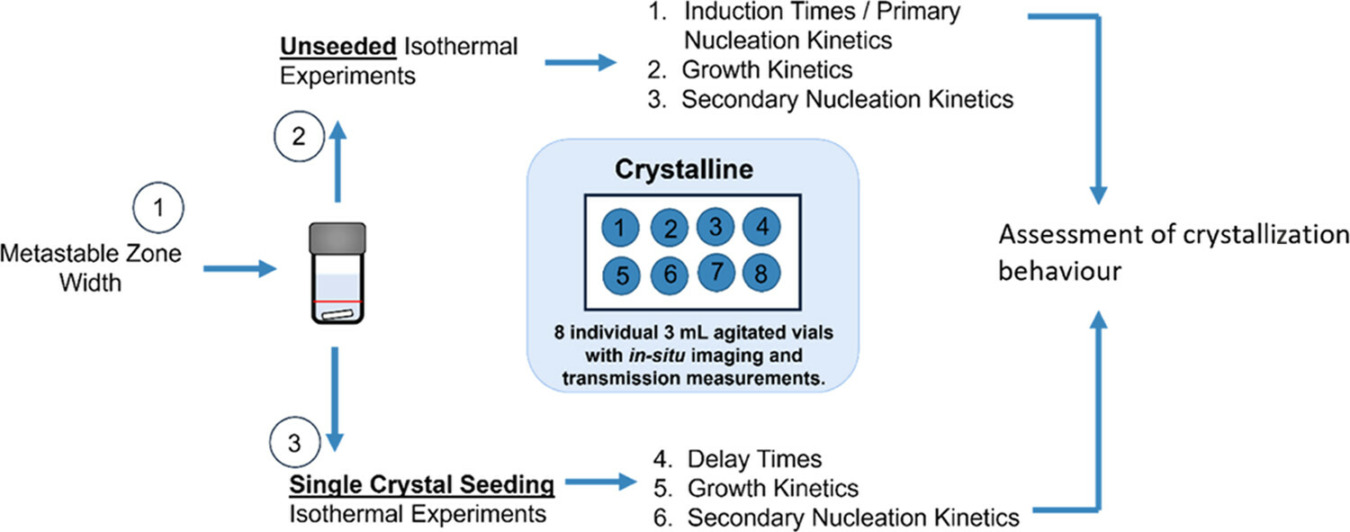
Overview of the workflow used to rapidly assess the crystallization behavior through estimation of primary and secondary nucleation and growth kinetics at given crystallization conditions (Cashmore et al.)
Similarly, taking advantage of Crystalline’s recent advances in technology, such as image analysis for crystallite counting and high throughput techniques for characterizing the highly stochastic nucleation process, Flannigan et al. [3] report on a detailed examination of the primary and secondary nucleation kinetics of NaCl, crystallized from solution, in water (H2O) and in the isotopologue D2O. They showed that crystallization conditions, especially sample agitation, have a very significant effect on crystallization growth kinetics by analysis of images from in situ cameras observing each sample, available in the Crystalline device only .
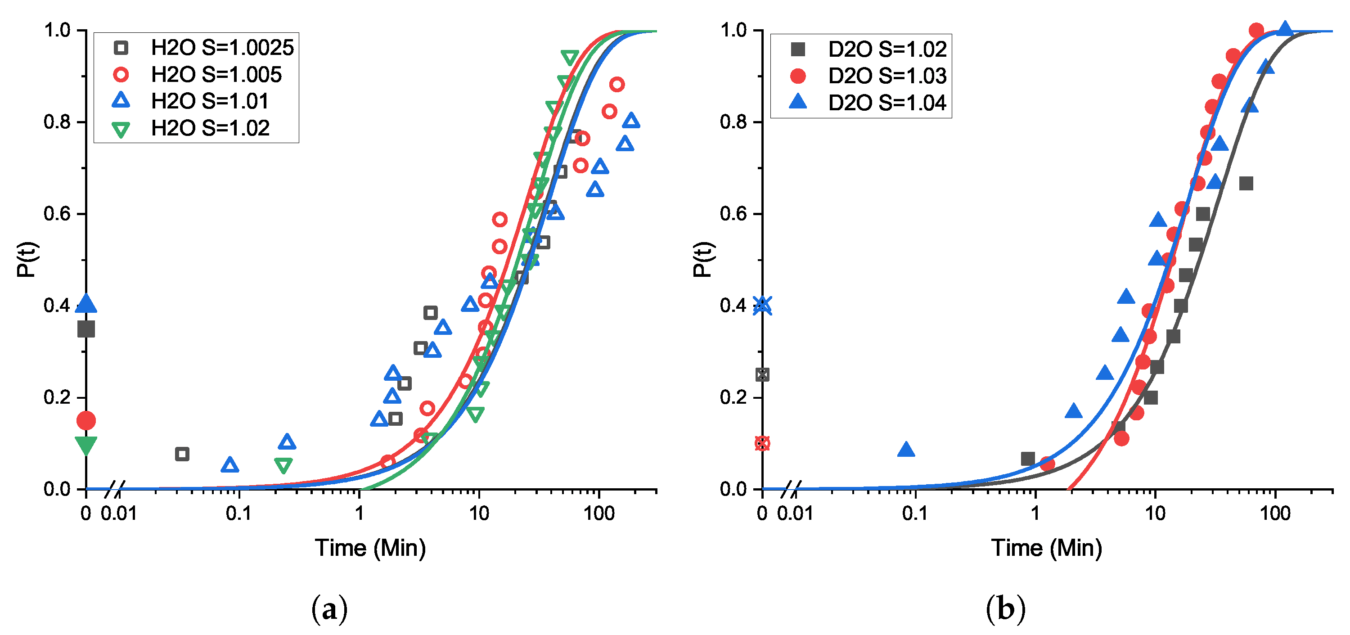
Cumulative probability distribution plots of induction time measurements for NaCl in H2O and D2O at 25 degrees C. Sample scale is 1g solvent, and agitation is by magnetic stirrer. Lines are fits to the Jiang and ter Horst model. (a) NaCl in H2O for supersaturations of 1.0025 (black open squares), 1.005 (red open circles), 1.01 (blue open triangles), and 1.02 (green open inverted triangles). (b) NaCl in D2O for supersaturations of 1.02 (black filled squares), 1.03 (red filled circles), and 1.04 (blue filled triangles). Filled symbols (a) and crossed symbols (b) on the t = 0 axis indicate the proportion of total vials that nucleated before reaching isothermal conditions: the P(t) points fitted are then proportions excluding these already-nucleated vials. Hence, for example, supersaturation > 1.02 could not be tested in H2O due to the overwhelming probability of nucleation occurring during the temperature quench before reaching the working temperature (see text) (Flannigan et al.)
Using Crystalline to determine temperature-dependent solubility curves
Employing particle engineering for crystalline surface coating on excipient core particles to improve the interactions with their environment, Zellnitz-Neugebauer et al. [4] tailored the surface roughness, topography as well as surface chemistry by manipulating the process settings and the choice of coating substance. Where the temperature-dependent solubility of α- and β-mannitol in the solvent was determined using the Crystalline instrument.
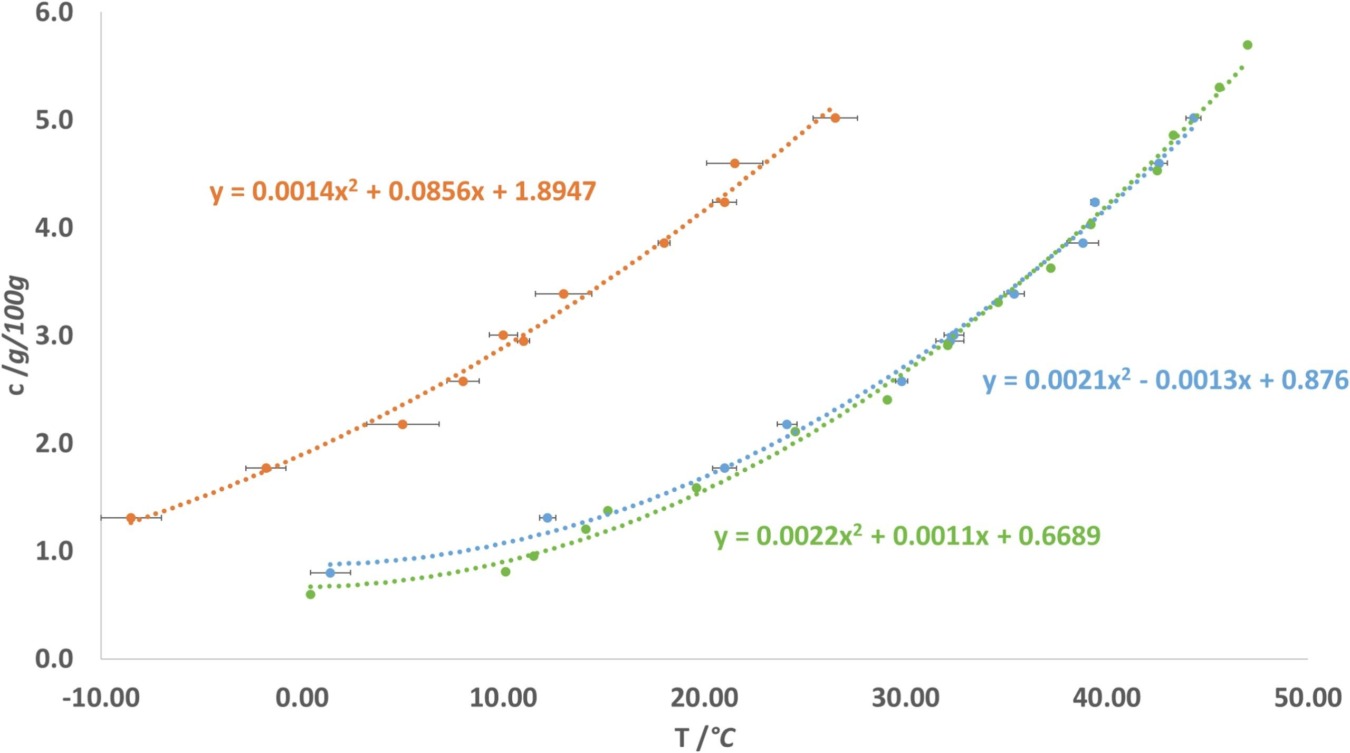
Solubility of α-mannitol (green) and β-mannitol (blue) in ethanol/water 60/40 (w/w) and metastable zone width of β-mannitol (orange) for a cooling rate of 0.25 °C/min. The dotted curves are representations of polynomic regressions of the data (Zellnitz-Neugebauer et al.)
Using Crystalline to track polymorphic form
Vélez et al. [5] adapted the hydrolysis–condensation reaction of TiO2 to the phase inversion temperature (PIT)-nanoemulsion method as a low energy approach to gain control over the size and phase purity of the resulting metal oxide particles. The nano-emulsion syntheses of the TiO2 particles were conducted in a Crystalline multi-reactor crystallization system. These syntheses were tested as a proof-of-concept in 8 mL reactors inside the Crystalline. Each reactor produced up to 150 mg of TiO2 per batch, with reproducible experimental yields ranging from 80 to 90%.
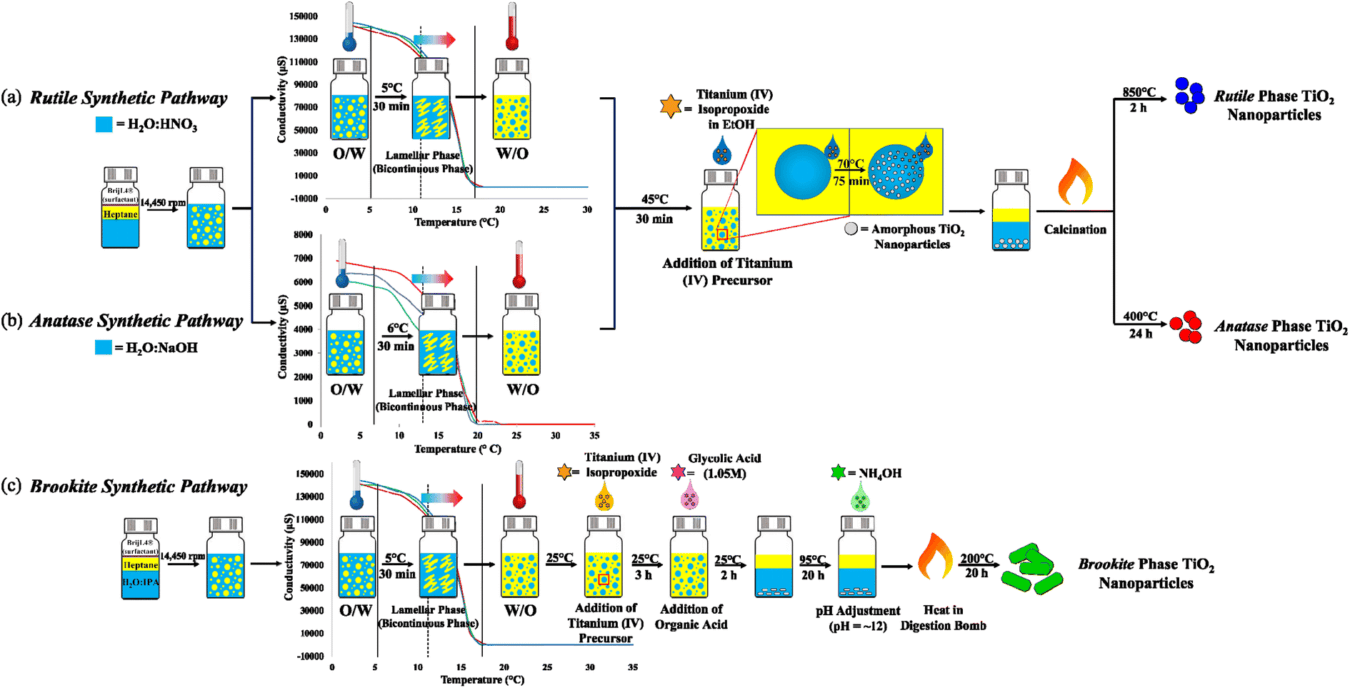
Schematic diagram of the PIT-nano-emulsion synthesis of (a) rutile, (b) anatase, and (c) brookite TiO2 highly-pure phase particles. The PIT determination graphs are inserted in each synthetic pathway, showing the inversion of phases at ∼11 °C for moderately and strongly acidic conditions (dashed line, light orange region) and ∼13 °C for alkaline conditions (dashed line, light pink region) (Vélez et al.)
Using Crystalline to study solution crystallization kinetics
Gerard Capellades' team at Rowan University [6] developed a high-throughput workflow using the Crystalline instrument for studying solution crystallization kinetics. This innovative approach combines automated batch crystallization experiments with advanced data analysis and parameter estimation techniques using PBM (Population Balance Model), as represented in the Figure 1. The Crystalline instrument was programmed to perform dissolution-crystallization cycles through controlled heating and cooling while capturing detailed imaging data during crystallization. Using the Crystalline instrument in this workflow highlights its significance in enhancing efficiency and precision in crystallization research with minimal time and raw material investment.
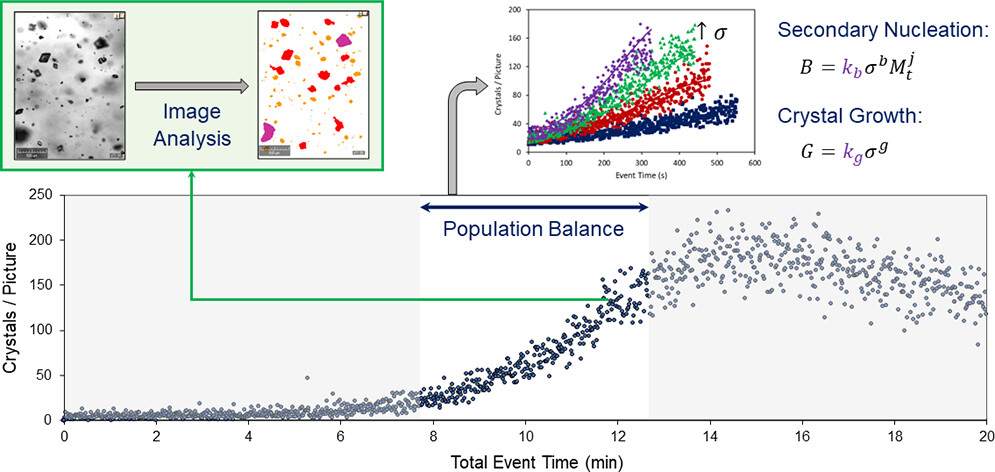
Figure 1. Graphical abstract of the research approach of the team at Rowan University
Using Crystalline as part of an autonomous DataFactory for the small-scale crystallisation of active pharmaceutical ingredients
Exploring future prospects, the CMAC Future Manufacturing Research Hub at the University of Strathclyde, Glasgow, UK [7], has effectively implemented a data-driven approach. By rigorously utilizing multiple Crystalline systems for image generation, the hub processes over 10,000 data points daily. The integration of robotic transfer facilitates continuous operation, 24/7, leading to a fourfold increase in throughput.
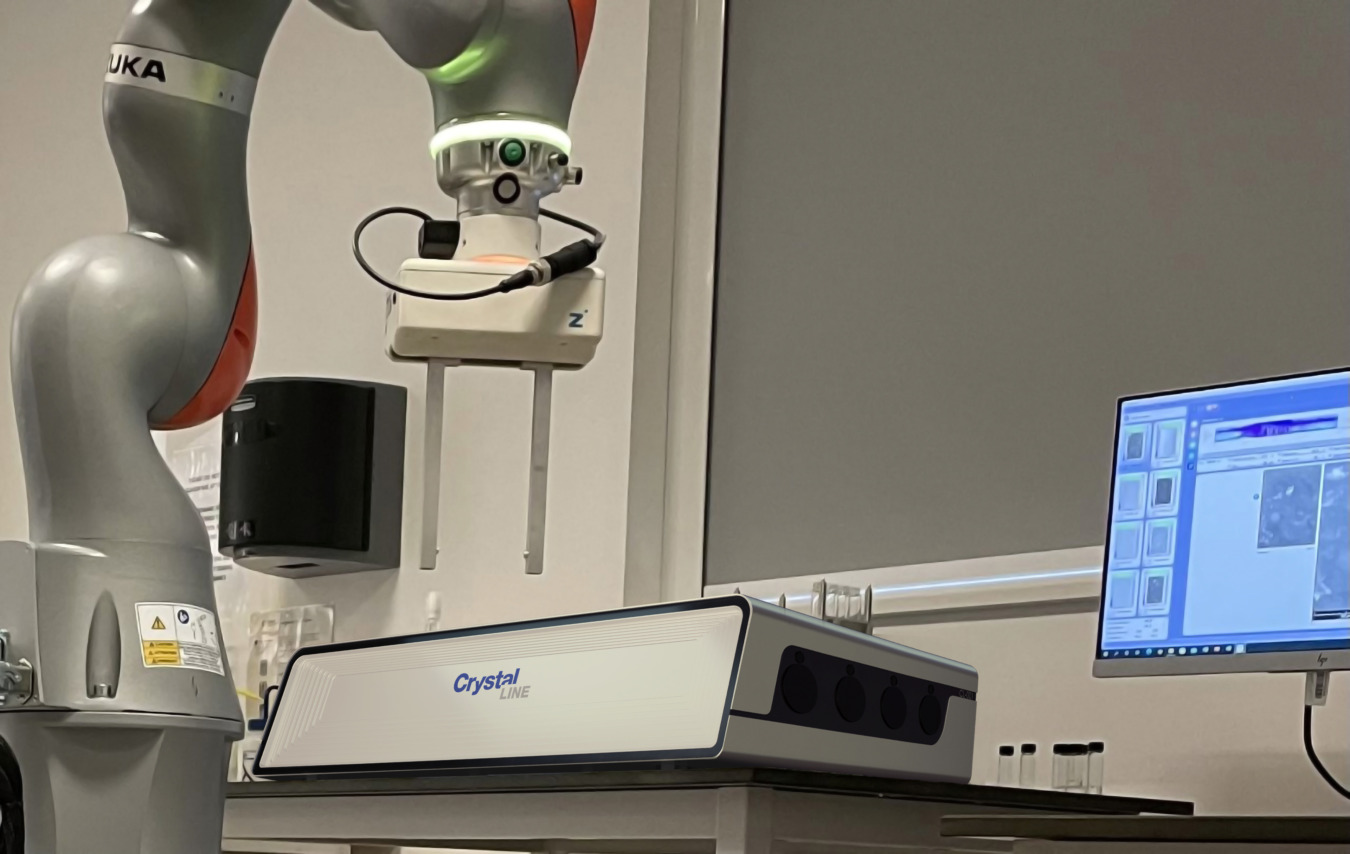
Crystalline instrument with robotic integration
References
- Nandi, S., Padrela, L., Tajber, L., & Collas, A. (2023). Nucleation kinetics-based solvent selection for the liquid antisolvent crystallization of a lipophilic intermediate. Journal of Molecular Liquids, 375, 121306.
- Cashmore, A., Miller, R., Jolliffe, H., Brown, C. J., Lee, M., Haw, M. D., & Sefcik, J. (2023). Rapid assessment of crystal nucleation and growth kinetics: comparison of seeded and unseeded experiments. Crystal Growth & Design 2023, 23, 7, 4779-4790.
- Flannigan, J. M., MacIver, D., Jolliffe, H., Haw, M. D., & Sefcik, J. (2023). Nucleation and growth kinetics of sodium chloride crystallization from water and deuterium oxide. Crystals, 13(9), 1388.
- Zellnitz-Neugebauer, S., Lanzinger, M., Schroettner, H., Naderi, M., Guo, M., Paudel, A., ... & Neugebauer, P. (2023). Temperature cycling-induced formation of crystalline coatings. International Journal of Pharmaceutics, 632, 122577.
- Vélez, G. Q., Nieves, D. S., Vázquez, A. C., & López-Mejías, V. (2023). Polymorphic control in titanium dioxide particles. Nanoscale Advances, 5(2), 425-434.
- Arruda, R. J., Cally, P. A., Wylie, A., Shah, N., Joel, I., Leff, Z. A., ... & Capellades, G. (2023). Automated and Material-Sparing Workflow for the Measurement of Crystal Nucleation and Growth Kinetics. Crystal Growth & Design, 23(5), 3845-3861.
- Poster presented at CMAC Open Days 2023, University of Strathclyde, Glasgow, UK, 14th Nov-16th Nov 2023. CMAC | CMAC Poster Books 2023; Poster no. 11.
See how Crystalline can help your research
Explore Crystalline's multiple applications to enhance your research.
Book a demo with our experts!